A central part our project is the analysis of lipid residues preserved in fragments from unglazed Roman period ceramic vessels, for example bowls, dishes, or jars. Lipids are mobilized from food items when these are stored and, particularly, cooked in the vessels and absorb into the porous ceramic matrix. This matrix can help to protect lipids from biotic and abiotic degradation and facilitate the survival of at least some lipids, even over many millennia. Because some lipids are more susceptible to degradation than others, the lipid patterns found in analysis are not identical to original ones, and extensive reference experiments are required to establish robust biomarkers and patterns to identify food items.
Sample preparation
The first step in our analytical workflow is the cleaning and sampling of sherds. To minimize the risk of contemporary contaminations (e.g., from handling in the field or museums), a small portion of sherd surface is abrasively cleaned before the section is separated from the sherd. As a general rule, we use about 2 g of ceramics (roughly the size of a pound coin). The sherd sections are then crushed to a powder with a mortar and pestle and (after addition of internal standards for quantification and quality control) extracted using a mixture of chloroform and methanol. This total lipid extract (TLE) is then used for preliminary screening as well as thorough characterization by high resolution mass spectrometry coupled to gas and liquid chromatography (GC-HRMS and LC-HRMS, respectively – see below). Finally, the lipids from aliquots are further converted to fatty acid methyl esters for stable isotope analysis (GC-C-IRMS).
Analytical techniques
For characterization of the extracted lipids we rely on chromatographic techniques coupled to powerful detectors. Gas chromatography (GC) is a superbly effective chromatographic technique, which allows for hundreds of constituents of complex mixtures to be separated from each other. This is achieved by exploiting differences in volatility and affinity to a chemically defined stationary phase within the separation column. In preliminary screening, GC is coupled to flame ionisation detection (GC-FID), which allows us to tentatively identify compounds by arrival time at the detector and produces a mass-flow dependent signal, thus allowing us to collect semi-quantitative data. When GC is coupled to (high resolution) mass spectrometry, additional molecular information can be collected to either verify the identification from screening or to deduce the structures of unknown compounds. In GC-MS, the compounds previously separated in the GC enter an ion source, where a stream of electrons leads to very reproducible fragmentation of the molecules. These fragments are subsequently individually detected and the patterns, just like puzzles, can then be used to identify the structures of the original molecule. These techniques are also extremely sensitive, allowing for the detection and characterization of picogram (10-12 g) amounts of molecules.
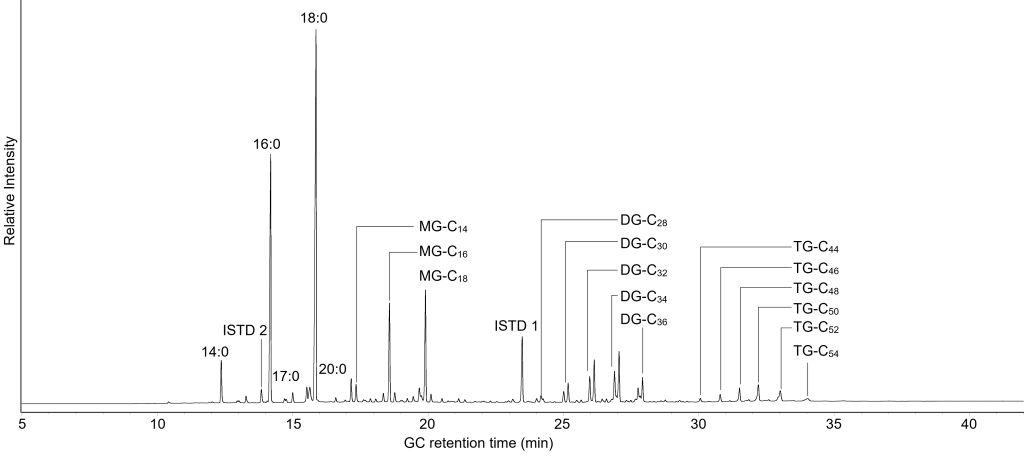
By nature of the technique, GC is very useful for separation of smaller and more volatile molecules, while larger molecules such as intact triacylglycerols can be analysed, but with some drawbacks. Similarly, some molecules are too polar or lack the volatility to be analysed by GC at all. Therefore, we are using a complimentary analytical approach based on liquid chromatography (LC) coupled to mass spectrometry as well. In LC the separation takes place in the liquid phase. The technique thus does not share the limitations of GC and can be used to collect additional, potentially diagnostic data. Although the mass spectrometers used in GC and LC differ slightly in their function and features, the approaches are very comparable, and the combination of both techniques will allow us to collect the most comprehensive data on preserved lipids achieved so far.
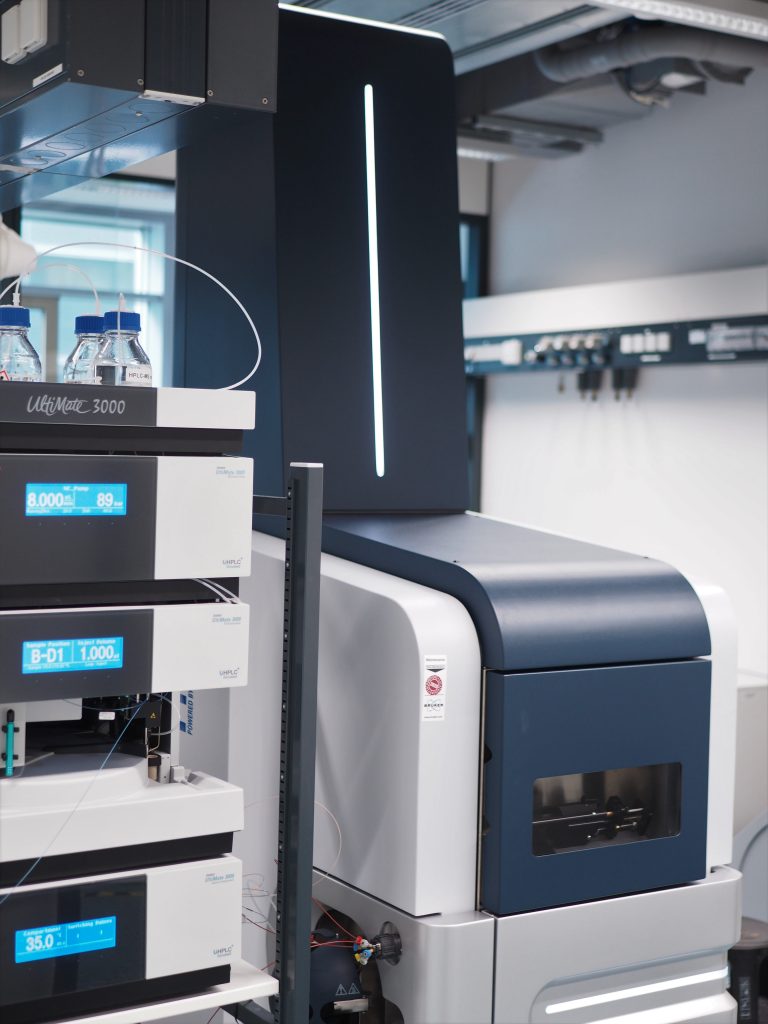
Lastly, to identify the major lipid sources we use GC coupled to isotope ratio mass spectrometry to collect compound-specific δ13C data for individual fatty acids. Here, the fatty acids from the GC are converted to CO2 in a combustion interface before the isotopologues (13CO2 and 12CO2) are detected individually by the mass spectrometer to calculate their ratios. Captured here are differences in the metabolic systems between ruminants and non-ruminants as well as routing of fatty acids between adipose and mammary tissues that lead to characteristic offsets between the δ13 values of palmitic acid (FA 16:0) and stearic acid (FA 18:0). Well established, this approach allows for the distinguishing of ruminant, non-ruminant and aquatic lipid sources as well as dairy from carcass lipids.